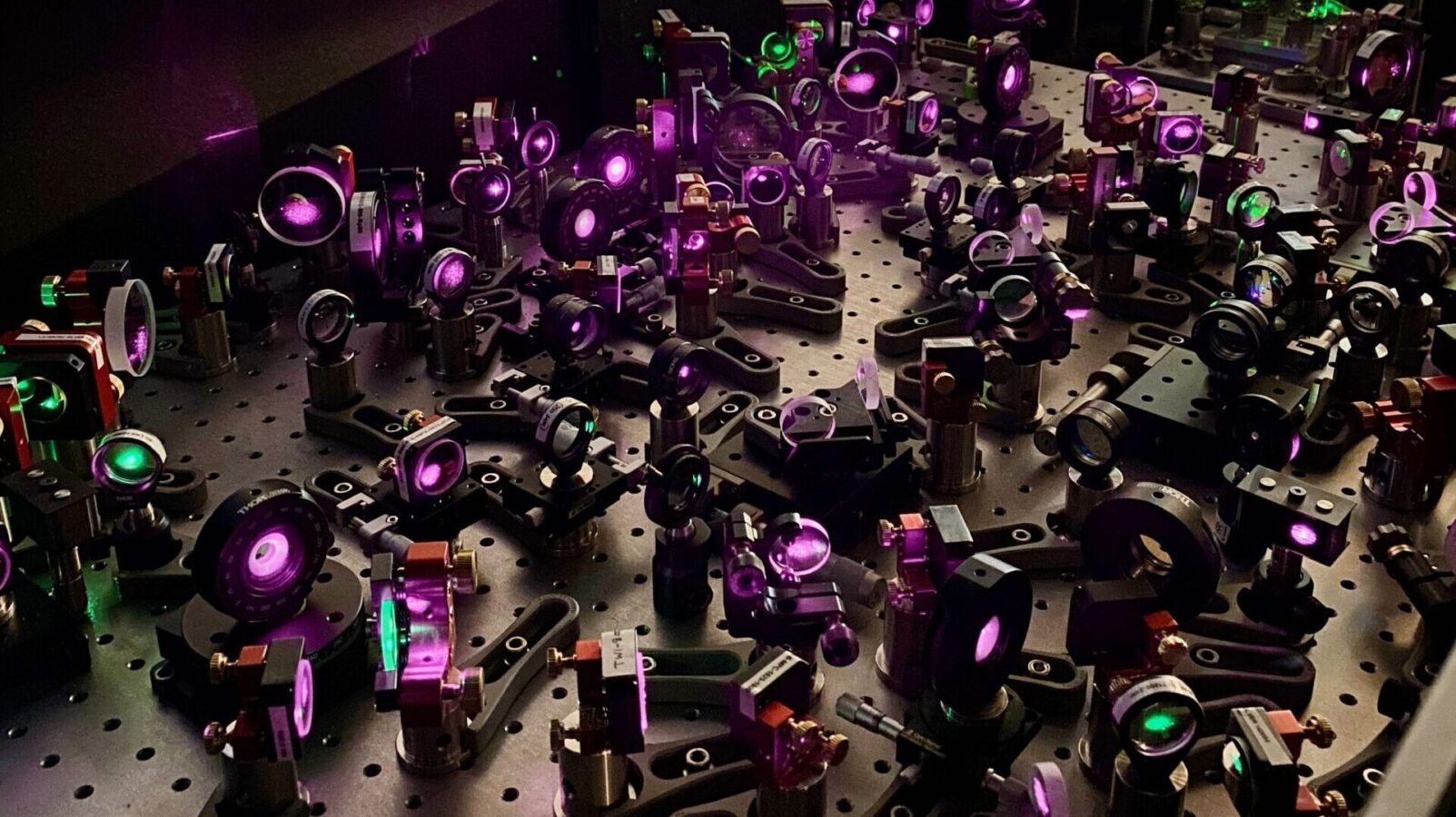
Home-built three-stage dual optical parametric amplifier system for generating highly tunable pulses in the mid-infrared and terahertz spectral ranges. Photo courtesy of MitranoLab (Harvard University).
In distinct classes of many-body systems, quantum coherence and entanglement persist across macroscopic length scales and a broad range of temperatures. This phenomenon is particularly evident in collective behaviors such as superconductivity, strange metallicity, or topologically ordered phases. However, the experimental validation and precise quantification of quantum correlations and entanglement in systems comprising a macroscopic (Avogadro) number of particles and degrees of freedom represents a current challenge.
In our group, we plan to address this question with the tools of ultrafast light-matter interactions. We develop the spectroscopic and theoretical methodologies to detect, isolate, and characterize quantum coherence on macroscopic scales. Additionally, we use techniques from non-equilibrium physics to engineer novel quantum phases that are beyond the reach of traditional thermodynamic pathways.
Strong-field quantum optics
In this line of research, we aim to explore the relationship between the quantum coherence of the probed system and the coherence of light during light-matter interactions. This entails the development of spectroscopic techniques capable of real-time sampling of photon correlation functions directly in the time domain, with sub-cycle precision, and rigorously map the resulting distributions to the matter quantum fluctuations and correlations, both in and out of equilibrium. Our emphasis will be on exploring non-linear responses, particularly through higher-harmonic generation experiments and multi-dimensional spectroscopies. These methodologies, distinguished by their highly non-linear nature, offer a unique sensitivity to nonlinear interactions, fluctuations, and disorder inherent to the system.
Simultaneously, we will further explore new strategies towards the generation of intense ultrabroadband quantum light exhibiting signatures such as anti-bunching, squeezing, and entanglement, to directly excite correlated many-body states inside complex materials. The ability to map the photon correlations onto matter and vice versa may provide a fresh perspective on many phenomena situated at the foundations of quantum electrodynamics, enable direct detection and manipulation of multipartite quantum states of matter with light, and ultimately lead to the discovery of new functionalities.
Periodically driven quantum materials
Ultrafast light-matter interaction enables accessing new phases of matter without thermal equilibrium counterparts. By developing pump protocols based on Floquet engineering with intense mid-infrared and terahertz excitation, our group will be exploring new pathways to suppress thermalization over an extended timescale and achieve the long-term stabilization of out-of-equilibrium phases of matter. The goal is to unveil intriguing phases that may include distinct non-equilibrium effects, previously elusive in real materials, such as eta-pairing condensation or many-body localization. This research represents a critical step towards translating achievements thus far confined to the realm of synthetic quantum matter, such as cold gases, into the domain of condensed matter systems.